Thank you for visiting nature.com. You are using a browser version with limited support for CSS. To obtain the best experience, we recommend you use a more up to date browser (or turn off compatibility mode in Internet Explorer). In the meantime, to ensure continued support, we are displaying the site without styles and JavaScript.
Scientific Reports volume 13, Article number: 15222 (2023 ) Cite this article Drying Oven Cabinet Dryer

Microcapsules of ciriguela peel extracts obtained by ultrasound-assisted extraction were prepared by spray drying, whose results were compared with those of freeze-drying as a control. The effects of spray-drying air temperature, feed flow rate and ratio of encapsulating agents (maltodextrin and arabic gum) were studied. Encapsulation efficiency, moisture content, total phenolic compounds (TPC), water activity, hygroscopicity, solubility, colorimetric parameters, phenolic profile by HPLC/DAD, simulated gastrointestinal digestion and morphology of spray-dried and freeze-dried microcapsules were evaluated, as well as their stability of TPC during 90 days storage at 7 and 25 °C. Spray-dried extract showed higher encapsulation efficiency (98.83%) and TPC (476.82 mg GAE g−1) than freeze-dried extract. The most abundant compounds in the liquid extract of ciriguela peel flour were rutin, epicatechin gallate, chlorogenic acid and quercetin. Rutin and myricetin were the major flavonoids in the spray-dried extract, while quercetin and kaempferol were in the freeze-dried one. The simulated gastrointestinal digestion test of microencapsulated extracts revealed the highest TPC contents after the gastric phase and the lowest one after the intestinal one. Rutin was the most abundant compound after the digestion of both spray-dried (68.74 µg g−1) and freeze-dried (93.98 µg g−1) extracts. Spray-dried microcapsules were of spherical shape, freeze-dried products of irregular structures. Spray-dried microcapsules had higher phenolic compounds contents after 90 days of storage at 7 °C compared to those stored at 25 °C, while the lyophilized ones showed no significant difference between the two storage temperatures. The ciriguela agro-industrial residue can be considered an interesting alternative source of phenolic compounds that could be used, in the form of bioactive compounds-rich powders, as an ingredient in pharmaceutical, cosmetic and food industries.
Stefano Nenciarini, Roberta Amoriello, … Duccio Cavalieri
Andrew Brodkorb, Lottie Egger, ... Isidra Recio
Arghya Mukherjee, Samuel Breselge, … Paul D. Cotter
In Brazil, it stands out the consumption of ciriguela (Spondias purpurea L.), a species belonging to the genus Spondias, which comprises more than 600 species concentrated mainly in the tropical regions of Africa, Asia and Central America1, 2. From a phytochemical point of view, ciriguela is rich in secondary metabolites, mainly phenolic compounds3, the main of which are gallic acid, kaempferol, quercetin and isorhamnetin4, 5. Thanks to their important antioxidant effects, phenolic compounds are beneficial to human health; they can in fact act as potential agents to prevent and treat diseases related to the oxidative stress and even to control cancer6, in addition to having proven antibiotic, antiallergic, anti-inflammatory and photochemical protection effects7. In addition, they can be used as substitutes for synthetic antioxidants as well as to produce functional foods, drugs and cosmetics8, 9.
The main by-products of fruit processing are peels and seeds, whose extracts contain a considerable amount of valuable substances with potential application in food, cosmetic and pharmaceutical industries10, 11. Compared to other extraction methods, ultrasound assisted extraction stands out, because it is a simple, green and low cost process that uses acoustic energy to improve the release and diffusion of target compounds from various matrices, makes less use of solvents and requires lower temperatures and shorter extraction time12,13,14. There are numerous limitations in the application of these extracts due to the instability of bioactive compounds under conditions of high temperature and presence of oxygen, light and enzymes, as well as pH variations15. These bottlenecks can be overcome by microencapsulation techniques, which are capable of increasing the stability of these compounds by protecting them from environmental adverse effects thanks to the incorporation of a protective matrix16, 17.
Among them, spray-drying, or atomization, is the most popular microencapsulation method18, 19. This technique, which consists of transforming a fluid suspension into dry particles, is widely used to encapsulate heat-sensitive food ingredients, such as bioactive compounds, using an encapsulating agent that acts as a coating material6, 17, 20. Commonly used encapsulating agents are biopolymers such as maltodextrin with different dextrose equivalents and arabic gum. Variations in the quantity and type of coating agent result in different encapsulation efficiencies and in powders with different physical and chemical properties21.
However, no studies have examined the effect of ultrasonic extraction of phenolic compounds on ciriguela waste, as well the influence of temperature, feed flow rate, and ratio of encapsulating agents on spray microencapsulation. Given the above, the main objective of the present work was to study the influence of temperature, feed flow rate and ratio of encapsulating agents (maltodextrin and gum arabic) on the microencapsulation by spray-drying of ciriguela waste extracts prepared by ultrasound-assisted extraction. It was also compared the TPC stability of the spray-dried extract with that of a control powder prepared by freeze-drying over 90-day storage at different temperatures (7 and 25 °C), for its possible use as a functional ingredient in food, cosmetic and pharmaceutical applications.
Ciriguela fruits were produced in the state of Paraíba (07° 09′ S 36º 49′ W). Ciriguela pulp by-products (peel and seed) were provided, after pulp extraction, by a frozen fruit pulp factory located in João Pessoa, PB, Brazil. After manual separation, 50 kg of ciriguela by-products yielded approximately 11 kg of ciriguela peel.
All chemicals used in this study were obtained from Sigma-Aldrich (St. Louis, MI, USA) or Merck (Darmstadt, Germany).
Ciriguela peel was subjected to drying at 60 °C for 24 h, according to Caldas et al.13, in an oven with air circulation and renewal (Model MA035/5, Marconi, Piracicaba, SP, Brazil) until reaching moisture below 10%. Then they were ground in a multipurpose mill (model TE 631/2, Tecnal, Piracicaba, SP, Brazil) and sieved through a 40-mesh (425 μm) screen. The resulting ciriguela peel flour (CPF) was stored in low-density 140-µm thick polyethylene bags, wrapped with laminated paper, and frozen to -20 °C for later analyses.
Soluble solids were determined with a digital refractometer (model r2i300, Reichert, Depew, NY, USA) and expressed in °Brix. The pH was analyzed using a pH-meter (HANNA, model HI 2210). The water activity (aw) was determined with a water activity meter (Decagon 4TE, Aqualab, Pullman, WA, USA) at 25 °C. The moisture content was determined on an infrared balance (model ID50, Marte Científica, São Paulo, SP, Brazil) at 105 ºC, and the results were expressed in %. Titrable acidity was determined titrimetrically, and the results were expressed in g of citric acid/100 g CPF22.
Ten g of CPF and 10 g ciriguela residue were separately extracted in 40 mL of solvent [80% (v/v) ethanol–water acidified with 0.1% (v/v) hydrochloric acid] by using a dynamic maceration, brought to room temperature (25 °C) and kept under stirring for 1 h. After extraction, suspended solids were removed by filtration through qualitative filter paper and the extracts obtained were stored at − 22 °C until further analysis.
The content of total phenolic compounds (TPC) in both in natura ciriguela residue and CPF was determined on a spectrophotometer (model UV-1650PC, Shimadzu, São Paulo/Brazil) at 725 nm using Folin-Ciocalteu reagent, according to the methodology described by Wettasinghe and Shahidi23. Briefly, 0.5 mL of each sample were incubated in a test tube with 8.0 mL of distilled water and 0.5 mL of Folin-Ciocalteu reagent. After 3 min of reaction, 1.0 mL of sodium carbonate solution was added, and the mixture was left to react for 60 min in the dark. The TPC content was calculated using a standard curve prepared from aqueous gallic acid solutions (0.1–1.0 mg/mL−1), and the results were expressed in mg of gallic acid equivalents (GAE) g−1 of CPF.
Ten g of CPF with 40 mL of solvent [80% (v/v) ethanol–water acidified with 0.1% (v/v) hydrochloric acid] were placed in a 100 mL beaker and subjected to the action of a ultrasonic probe (model QR1000 Eco-sonic, Ultronique, Sao Paulo, SP, Brazil), using 1000 W power, 20 kHz frequency and 15 min exposure time24. The extracts obtained were filtered and kept in the absence of light at − 20 °C for later analyses.
Microencapsulation by atomization was performed in a spray dryer (model MSD 1.0, Labmaq do Brasil, Ribeirão Preto, SP, Brazil). The carrier agents used were maltodextrin 10DE (Ingedion, Mogi-Guaçu, SP, Brazil) and gum arabic (Dinâmica Química Contemporânea, Indaiatuba, SP, Brazil). Extracts together with the encapsulating agent formulation were homogenized in Turrax (model TE-102, Tecnal—Piracicaba, São Paulo/Brazil) using a speed of 14,000 rpm for 5 min. The content of total solids in the suspension (wall material plus extract) was fixed at 30%, and atomization was performed using 1.2 mm diameter injector nozzle, 30 m3 h−1 air flow rate and 0.6 bar air pressure.
Runs were carried out according to a 23-full experimental design composed of 8 factorial points (levels ± 1) and 3 central points (level 0), totaling 11 runs (Table 1).
Temperature (T), ratio of encapsulating agents (F) and feed flow rate (V) were the independent variables, while moisture content, water activity, hygroscopicity, solubility, TPC content, encapsulation efficiency and chromatic parameters were the response variables. The data obtained were adjusted to Eq. (1):
where Y is the response, β0 is the constant regression coefficient, β1, β2 and β3 are the linear coefficients, T, F and V are the independent variables, and β4, β5, β6 and β7 are the coefficients of the interaction effects TF, TV, FV and TFV, respectively.
Microencapsulation by freeze-drying, used as a control, was performed for 48 h in a lyophilizer (model Alpha 1–4 LD Plus, Martin Christ, Osterode am Harz, Germany) at − 80 °C and 0.28 mbar chamber pressure, using the optimum encapsulating agent’s formulation established for microencapsulation by spray-drying.
These characteristics of the microencapsulated extracts were determined by the same methodologies used for in natura ciriguela residue and CPF.
The hygroscopicity of microencapsulated extracts was determined according to the methodology described by Cai and Corke25 with some modifications. Briefly, one g samples of each microencapsulated extract were placed at 25 °C in an airtight container containing a saturated NaCl solution (75.29% relative humidity) and weighed after one week. Hygroscopicity was expressed in g of moisture absorbed per 100 g of sample dry mass (g/100 g).
The solubility in water of microencapsulated extracts was determined according to the methodology reported by Cano-Chauca et al.26. To this purpose, 1.0 g samples of each powder were suspended in 100 mL of distilled water, stirred for 5 min in a magnetic stirrer (model 752, Fisatom, São Paulo, SP, Brazil) and centrifuged at 3000 rpm (1800 × g) for 5 min in a centrifuge (model CT-6000R, Cientec, Belo Horizonte, MG, Brazil). A 25-mL supernatant aliquot was placed in a pre-weighed sterilized Petri dish and kept at 105 °C for 5 h in oven. The plate was then weighed on an analytical balance, and the solubility determined by weight difference.
The chromatic parameters of microencapsulated extracts were evaluated with a colorimeter (model CR 400, Konica Minolta, Sensing Inc., Osaka, Japan), using the color standards of the Commission Internationale de L'Eclairage (CIELab) system, namely, luminosity (L*) ranging from white (100) to black (0), intensity of the green (− a*) to red (+ a*) component of light, and intensity of the blue (− b*) to yellow (+ b*) component.
The variation in color (ΔE*) compared to the in natura extract was calculated by Eq. (2)25:
where L0* and L*: are the luminosities of the samples of the free extract and the reconstituted microencapsulated extract, respectively; a0* and a*: are the red to green color intensities of the free extract and reconstituted microencapsulated extract samples, respectively; b0* and b*: are the intensities of the yellow to blue color of the samples of the free extract and the reconstituted microencapsulated extract, respectively.
The phenolics contents in microencapsules (TMPC) and on microcapsules surface (SMPC) were determined spectrophotometrically at 725 nm according to Saénz et al.27, after reaction with the Folin-Ciocalteu reagent and using a gallic acid standard curve23. The results were expressed in mg of gallic acid equivalents per g of microcapsules (mg GAE/g−1).
The microencapsulation efficiency (ME) was calculated by Eq. (3) according to Mahdavi et al.28:
The apparent density of microcapsules (ρap) was determined according to the procedure described by Barbosa-Cánovas and Juliano29 and Caparino et al.30 with some modifications. Two g of sample were transferred to a 10-mL graduated test tube, where the powder was compacted by beating it 50 times on the bench. ρap, expressed in g/mL, was calculated according to Eq. (4):
where m is the sample mass (g) and V the total volume occupied by the powder in the tube (mL).
The absolute density (ρabs) was determined at 25 °C by means of a pycnometer provided with thermometer according to the methodology proposed by Caparino et al.30 and expressed in g mL−1.
The intragranular porosity (ε) was calculated according to Caparino et al.30 using Eq. (5):
The 1,1-diphenyl-2-picrylhydrazyl radical (DPPH.) scavenging capacity of microcapsules was assessed according to the method described by Brand-Williams et al.31 and modified by Sánchez-Moreno et al.32. The extract was diluted up to three different TPC concentrations by its addition to a 0.1 M DPPH: solution in methanol. The absorbance at 517 nm was monitored with a spectrophotometer (model UV-1650PC, Shimadzu, São Paulo, SP, Brazil) until the reaction reached a plateau. The results were expressed according to Ramadan et al.33. The inhibition percentage (IC50), i.e., the sample concentration needed to inhibit the DPPH• radical formation by 50%, was obtained according to Eq. (6):
where AS is the absorbance of the sample suspension and ADPPH is the absorbance of the DPPH solution.
The sample concentration providing IC50 was calculated by interpolation from the graph of radical-scavenging activity percentage against sample concentration.
The ferric reducing antioxidant power (FRAP) of microcapsules was determined by monitoring the absorbance at 593 nm, and the results were expressed as µmol of ferrous equivalent per g of powder (µmol Fe2+ g−1)34.
One g sample of microencapsulated extract was added to 10 mL of 6.0 M HCl in methanol and submitted for 30 min to extraction under ultrasonication at 25 °C and 40 kHz (model USC-1800, Unique, Indaiatuba, SP, Brazil). The extract was then centrifuged at 3000 × g for 20 min (model SL-701, Solab, Piracicaba, SP, Brazil). A 1.0-mL aliquot of the supernatant was filtered through a polytetrafluoroethylene syringe filter with 0.45-µm pore diameter and used to identify and quantify the phenolic compounds by High-Performance Liquid Chromatography (HPLC).
The methodology validated by Padilha et al.35, with adaptations by Dutra et al.36 on gradient and runtime, was used to quantify individual phenolic compounds using an Agilent 1260 Infinity LC System (Agilent Technologies, Santa Clara, CA, USA) coupled to a diode arrangement detector (DAD) (model G1315D). Separation of phenolic compounds was performed in a Zorbax Eclipse Plus RP-C18 column (100 × 4.6 mm, 3.5 µm) and a Zorbax C18 precolumn (12.6 × 4.6 mm, 5 μm). Data collection and analyses were carried out using the software OpenLAB CDS ChemStation Edition (Agilent Technologies). After dilution in solvent A (0.1 M phosphoric acid solution, pH 2.0), samples were filtered through a membrane with 0.45-μm pore diameter (Millex Millipore, Barueri, SP, Brazil) and injected (20 μL). The oven temperature was 35 °C, the eluent a mixture of solvents A and B (metanol acidified with 0.5% phosphoric acid), and the eluent flow rate 0.8 mL min−1. The gradient used in the separation was 0–5 min: 5% B, 5–14 min: 23% B, 14–30 min: 50% B, 30–33 min: 80% B. Detection of compounds was done at 220, 280, 320, 360 and 520 nm, and the identification and quantification by comparison with external standards. The results were expressed in µg g−1 dry weight.
The gastrointestinal digestion simulation was performed, according to Rodrigues-Roque et al.37 and Dutra et al.4, mimicking the physiological gastrointestinal conditions, i.e., considering two sequential phases in stomach (gastric) and small intestine including dialysis (intestinal). Microencapsulated extract aliquots (50 mL) were mixed with 5 mL of simulated salivary solution (2.38 g Na2HPO4, 0.19 g KH2PO4, 8 g NaCl, and 200 U/L of α-amylase) in amber vials. After homogenization of the mixture for 10 min in a water bath at 37 ± 1 °C and 95 × g, the samples were acidified to pH 2.0 with 1.0 mL of a porcine pepsin solution (13 mg pepsin in 5 mL of 0.1 M HCl) and later incubated at 37 °C under agitation at 95 × g for 1 h to simulate gastric digestion. The mixture was then immediately cooled in an ice bath, and a 1.0-mL aliquot was stored at − 18 °C, while the rest of the sample was submitted to intestinal digestion. Thirty cm long dialysis membrane segments were filled with 25 mL of a 0.5 M NaHCO3 solution, which was used to titrate the gastric digestate at pH 7.5. Each 20-mL sample of gastric digestate was placed in a polyethylene tube, and a dialysis membrane was completely immersed until pH 5.0 was reached. Then, 5.0 mL of pancreatine (0.12 g) and bile salts solution (40 mg glycodeoxycholate, 25 mg taurodeoxycholate, 40 mg taurocholate in 1.0 mL of saline) were added to each tube. The samples were incubated under agitation of 95 rpm at 37 °C for 2 h to complete the intestinal phase. Finally, the dialysis membrane was removed and rinsed with distilled water. The bioaccessible fraction of phenolic compounds transferred within the dialysis membrane was then analyzed by HPLC to determine the profile of residual phenolic compound after simulated gastrointestinal digestion.
The average diameter and particle size distribution were determined on a laser diffraction analyzer (model S3500, Microtrac, Largo, FL, USA) coupled to a common bench ultrasound device to increase sample dispersibility. A small amount of sample was dispersed in isopropyl alcohol as a carrying fluid and subjected to particle size distribution readings. The average diameter was determined based on the average diameter of a sphere of the same volume (Brouckere diameter, D[4.3]), which is generally used to characterize powder particles.
The morphology of microparticles was examined with a scanning electron microscope (SEM) (model Vega 3, Tescan, Brno, Czech Republic). The samples were fixed in metallic specimen holders (stubs) with a conventional electrically conductive double-sided adhesive tape, metallized with gold in a metallizer (model EM SCD500, Leica, Wetzlar, Germany) at a coating rate of 15 nm thickness for 80 s and a current of 40 mA, and examined with the above SEM operating at 10 kV. Image acquisition was performed using the XT microscope software.
Microcapsules were stored according to Nunes et al.38 with some modifications. The samples (1.0 g) were placed in flexible laminated packages (Zip lock), kept at two different temperatures (7 and 25 ± 1 °C) and stored for 90 days. The TPC content was determined at 0, 15, 30, 45, 60, 75 and 90 days.
Experimental data were analyzed and presented as means plus standard deviations of triplicate measurements. Analysis of variance (ANOVA), F-test for lack of fit, determination of regression coefficients and generation of response surfaces were done with the aid of Statistic 7.0 software (Statsoft, Tulsa, OK, USA) at 5% error probability.
Table 2 lists the results of the physicochemical characterization of in natura ciriguela residue and ciriguela peel flour (CPF).
The waste drying process resulted in a total solids content in CPF within the range found by other authors for flours of different fruit residues39, 40. The pH values of both in natura ciriguela residue and CPF are in the range of acid foods, which suggests inhibition of the growth of most pathogenic and deteriorating microorganisms. This value is in agreement with that reported by Albuquerque et al.39 (3.17) for ciriguela whole flour. The water activity (aw) of CPF (0.178 ± 0.004) was much lower than that of in natura residue, which indicates that it can be considered microbiologically safe41.
Table 3 shows the results of the quality parameters (water activity, moisture content, hygroscopicity and solubility) of the CPFs prepared by spray-drying according to the experimental design shown in Table 1.
The results of analysis of variance (ANOVA) applied to linear models (Eq. 1) of each response variable, omitting the non-significant terms, are gathered in Table 4.
As is known, aw, moisture content and hygroscopicity are important characteristics for the stability and storage of any powder, while solubility is associated with its eventual reconstitution. All aw values of spray-drying microencapsulated extracts (0.155–0.235) (Table 3) were lower than the threshold limit (0.3) below which powders can be considered stable and resistant to the attack of both spoilage microorganisms and enzymes responsible for lipid oxidation42, 43. aw values close to those obtained in this study were reported for different bioactive compounds-rich microencapsulated extracts19, 44,45,46,47.
This response was significantly influenced by inlet air temperature (T), interaction between temperature and feed flow rate (TV) and interaction between the ratio of encapsulating agents and feed flow rate (FV), according to Eq. (7):
The higher temperature, the lower the aw value of powder extracts, which indicates its positive effect on this response, similar to that observed by Tülek et al.48 for atomized lemongrass extract. When using a high feed flow rate of (0.80 mL min−1) and combined with 100% gum arabic as an encapsulating agent, the extracts showed lower aW, probably due not only to the influence of the FxV and TxV binary (p < 0.05) (Figs. 1S and 2S of the Supplementary Material), but also the ability of gum arabic to incorporate water molecules thanks to the presence of proteins and hydrophilic groups in its composition19. Similarly, Bednarska and Janiszewska-Turak49 and Janiszewska-Turak et al.43 observed that the greater the addition of gum arabic, the lower the aw values of chokeberry and carrot powder juices, respectively.
Moisture contents in the 1–6% range are sought by industry to ensure stability of powder products during storage42. All moisture contents obtained in this study, varying from 3.16 to 5.58% (Table 3), fell within this range and were close to those reported in several studies for different atomized fruit extracts or by-products, such as cagaita (Eugenia dysentrica DC)50, grape (Vitis labrusca var. Bordo) skin45 and grape pomace51, acerola residue47, pineapple peel52, maca leaf16, cranberry juice19, cocoa pod husk46, and mulberry (Morus alba L.) leaf6.
Moisture content was significantly influenced by the linear effects of T and F as well as the ternary interaction between T, F and V (p < 0.05) (Tables 3 and 4), according to Eq. (8):
ANOVA showed a coefficient of determination (R2) of 0.87 (Table 4), indicating that fitting of experimental data to this model was more than satisfactory. In particular, the decrease in microcapsule moisture content resulting from an increase in air inlet temperature (Figs. 3S of the Supplementary Material). The temperature had a negative effect on the moisture of the powders, higher inlet air temperature (170 °C) produced extracts in powders with lower moisture contents, probably due to different drying rates between the droplets of the feed solution and the air of drying due to their different physical properties52. The same negative effect of air temperature on moisture content has been reported for different phenolic compounds-rich atomized extracts50,51,52,53. According to Kaderides et al.54, the higher the temperature difference between drying air and particles, the higher the heat transfer rate to the particles, thus causing a driving force for moisture removal. The powders produced with gum arabic as an encapsulating agent exhibited the highest moisture contents and (Fig. 3S of the Supplementary Material), confirming the trend observed by Pudziuvelyte et al.18 for Elsholtzia ciliata essential oil microcapsules. According to Tran and Nguyen55, gum arabic has higher capacity of absorbing water from surrounding environments than maltodextrin.
The hygroscopicity of powders, varying from 11.20 to 15.24 g of water absorbed/100 g of sample (Table 3), was low, which promises to facilitate their conservation and preserve their color and content of bioactive compounds. This response variable was significantly influenced by T, F, V and ternary TxFxV interaction (p < 0.05) (Tables 3 and 4), according to Eq. (9):
Hygroscopicity values close to those of this study were reported for different spray-dried extracts44, 45, 47, 50. More hygroscopic powders were produced when higher feed rates were used. This response was influenced mainly and positively by V and negatively by F (Figs. 4S, 5S and 6S of the Supplementary Material). Similar results were obtained for chokeberry juice powder49.
Water solubility of atomized extracts, ranging from 86.43 to 92.20% (Table 3), fell within the solubility range reported for other atomized fruit extracts or by-products18, 44, 47, 52. It was significantly influenced by F and by the FxV and TxV binary interactions (p < 0.05), according to Eq. (10):
This model, however, was not predictive due to a too low R2 value (0.5) (Table 4), so the effect of each variable on powders solubility were illustrated in the form of Pareto Graph (Fig. 7S of the Supplementary Material). The maltodextrin/gum arabic ratio in the coating agents formulation had a positive effect on water solubility, in that, powders produced with maltodextrin were more soluble, although the solubility of all them was high (Fig. 8S of the Supplementary Material). The high solubility of powders produced in this work can be related to the high solubility of the selected encapsulating agents, as well as the product granulometry, in that, the lower the particles size, the greater the surface area available for hydration45, 56. As shown in Fig. 9S (Supplementary Material), the most soluble microencapsulated extracts were those produced using the lowest feed flow rate and maltodextrin as a wall material.
The microencapsulation efficiency (ME) of atomized extracts ranged from 76.83 to 99.84% (Table 3), indicating that the selected formulation of encapsulating agents was very efficient in protecting phenolic compounds. This response was significantly influenced by all independent variables and their combinations (p < 0.05) according to Eq. (11):
The model had statistical and predictive significance with R2 of 0.97. When the value of pure error is low as in this case, i.e., the reproducibility is very good, the lack of fit, even though significant (Table 4), is a false result due to the fact that the F-calculated value is high because of the very low denominator value. This model was validated through ANOVA before building the response surface charts illustrated in Fig. 1a–c.
Response surfaces of the efficiency of ciriguela residue extracts encapsulated by spray-drying as a function of (a) temperature and ratio of encapsulating agents; (b) feed flow rate and ratio of encapsulating agents; and (c) feed flow rate and temperature.
The spray-dried extracts produced with gum arabic showed the highest ME values, in agreement with the results obtained in microcapsules of E. ciliata essential oil55. It has been reported that ME of microencapsulated extracts would be related to the combination of encapsulating agents used, which would be responsible for different filmogenic properties, keeping the active principle protected45.
The TPC content of spray-dried extracts, which ranged from 190.45 to 454.66 mg GAE g−1 (Table 3), was significantly influenced by F, V, TF, TV, and FV (Table 4) according to Eq. (12):
The high R2 value (0.98) compensates for the significant lack of fit for the same reasons as for ME (Table 4), indicating that the model has statistical and predictive significance. The influence of independent variables on this response is illustrated by the response surfaces in Fig. 2a and b.
Response surfaces of the total phenolic compounds (TPC) content of ciriguela residue extracts encapsulated by spray-drying as a function of (a) ratio of encapsulating agents and feed flow rate and (b) ratio of encapsulating agents and temperature.
Since the ratio of coating agents in the encapsulating formulation was the variable that most influenced this response, followed by the FV interaction, the highest TPC contents were obtained using gum arabic (Fig. 2a and b). A similar result was reported for the spray-drying of grape (V. labrusca var. Bordo) skin extract45. This special gum arabic capacity of encapsulating TPC has been related to its structure, being a highly branched sugar heteropolymer with small protein content, which allows protection of these compounds mainly during the critical early phase45, 47. As a result, all the runs performed using the mixture of encapsulating agents (9, 10, and 11) also led to TPC contents higher than those obtained only with maltodextrin, similar to what was observed by Rezende et al.47 for atomized acerola residue extract.
According ANOVA applied to results from runs performed according to the above experimental design, the optimal conditions for microencapsulation by spray-drying of CPF extracts were a temperature of 150 °C, a feed flow rate of 0.80 L h−1 and 100% gum arabic as a coating agent. Microencapsulation by freeze-drying, used as a control, was performed with the same encapsulating agent. Table 5 lists the results of physicochemical parameters and antioxidant activity of ciriguela residue extracts microencapsulated by both drying methods.
ME, TPC content, antioxidant activity by the DPPH method, apparent density and intragranular porosity showed statistically significant differences (p < 0.05) between spray-dried and freeze-dried extracts, while antioxidant activity by the FRAP method did not (p > 0.05) (Table 5). These results suggest the importance of using different methods for the safe and conclusive determination of antioxidant activity, as each method has its own specificities and acts better in a specific field47. Higher values of ME, TPC content and antioxidant activity by DPPH were obtained for the spray-dried extract (p < 0.05). On the other hand, Ramírez et al.57, when studying the stability of freeze-dried and spray-dried fruits, observed greater retention of polyphenols in the former encapsulates.
Luminosity (L*) values were close to 90.00 (Table 5), indicating that spray-dried and freeze-dried extracts had color close to white, similarly to what was observed for spray-dried cagaita extract50. According to Comunian et al.58, these results are due to the dilution effect of the white encapsulating agents. Although microencapsulation by spray-dring, due to high inlet air temperature, can lead to darkening reactions responsible for color loss59, the intensity of green/red component of light (a*) and difference in color (ΔE*) showed no significant difference between the two extracts, unlike the intensity of yellow/blue component of light (b*) (p < 0.05). Taking the colorimetric parameters as a whole (L*, a*, b*), it can be concluded that the CPF extract microcapsules can be considered of greenish-yellow color like those obtained from mulberry (M. alba L.) leaf6.
Physical parameters showed no significant differences between spray-dried and freeze-dried extracts (p > 0.05), except for apparent density and intragranular porosity that were 24% higher (0.31 ± 0.01 g mL−1) and 8.6% lower (64.37 ± 0.95%), respectively, for the freeze-dried extract (Table 5). It is noteworthy that these characteristics play an important role in controlling the degree of rehydration and reconstitution of powders.
The profile of phenolic compounds was investigated by HPLC–DAD in both the liquid extract and the microcapsules prepared by spray-drying and freeze-drying using 100% gum arabic as a coating agent (Table 6). Phenolic acids, flavanols and flavonols were identified as the four major groups, while flavanones, stilbenes and anthocyanins were detected in lower amounts. Although the contents of identified phenolics varied statistically between the two powders (p < 0.05), as expected, their qualitative profile (Table 6) was relatively similar to that observed for frozen ciriguela pulp4 and ciriguela peel60, 61. This shows that after microencapsulation of ciriguela residue extract, the microcapsules still had phenolic compounds with biological activities of interest such as antioxidant, antimicrobial and anti-inflammatory attributed to the various phenolic compounds found4, 62.
Rutin (342.59 ± 45.08 µg g−1), epicatechin gallate (228.63 ± 12.78 µg g−1), chlorogenic acid (228.31 ± 5.67 µg g−1), and quercetin (181.02 ± 6.28 µg g−1) were the most abundant phenolic compounds in the CPF liquid extract. Chlorogenic acid was the only phenolic acid found in spray-dried (40.09 ± 1.53 µg g−1) and freeze-dried (17.72 ± 0.17 µg g−1) extracts. Catechin content was significantly higher (p < 0.05) in the freeze-dried extract (93.94 ± 0.67 µg g−1) compared to both liquid and spray-dried extracts (Table 6). Isorhamnetin was present only in the spray-dried sample, while epicatechin, epigallocatechin gallate, and hesperidin were only detected in the liquid and spray-dried extracts. Rutin was the major flavonol in both liquid (342.59 ± 45.08 µg g−1) and spray-dried (82.69 ± 0.70 µg g−1) extracts, while kaempferol was in the freeze-dried one (70.46 ± 1.33 µg g−1). Santana Neto et al.63 observed a similar phenolic acids profile to that of this study in extract ciriguela residue, and among the flavonoids identified, rutin had the highest level.
trans-Caftaric acid, caffeic acid, p-coumaric acid, trans-resveratrol, and petunidin 3-glucoside were found only in the liquid extract. In this regard, it has been reported that when fruits are subjected to processing, including maceration, crushing, microencapsulation, or freezing, oxidation and/or degradation of antioxidant compounds may occur4, 64,65,66.
The contents of phenolic compounds recovered after each phase (oral, gastric, and intestinal) of simulated gastrointestinal digestion of microencapsulated extracts are listed in Table 7. It is noteworthy that the higher this residual content, the greater the resistance of microcapsules to gastric conditions. The highest values were found after the gastric phase (p < 0.05), while the minor ones after the latter, i.e., the intestinal one. A similar result was reported for flour from persimmon (Diospyros kaki) fruit co-product during in vitro gastrointestinal digestion67.
While in the post-gastric phase most phenolic compounds were identified, in the post-intestinal phase there was a significant reduction in the number of compounds. This tendency may be related to digestion conditions, mainly due to changes in gastric pH (pH 2.0) and intestine (pH 7.5)68. The acidic conditions of the gastric phase probably contributed to the release of the compounds.
During gastric digestion, several phenolic acids, flavanols, and flavonols were detected, highlighting the significant phenolic content available for absorption. However, more research is needed to investigate the dynamics of the absorption of phenolic compounds in the stomach. The transition from gastric to intestinal digestion reduced the bioaccessibility of most compounds, but did not increase the bioaccessibility of procyanidin A2 from the atomized extract.
Regarding the microcapsule production method, freeze-drying ensured greater phenolic compound content than spray-drying, except for epigallocatechin gallate and quercetin. Most phenolic compounds were completely degraded after the gastric phase, except for epicatechin gallate and rutin in the freeze-dried extract, while procyanidin A2 was detected only after the intestinal phase in the spray-dried one. Finally, the highest concentrations of epicatechin gallate and rutin (p < 0.05) were detected after the gastric phase, similar to what was observed for persimmon fruit co-product flour67.
A total of three compounds were detected after the intestinal phase of digestion and six were not detected. This finding is consistent with several studies that found a considerable decrease in phenolic compounds after gastrointestinal digestion67, 69, 70. The loss of these compounds may have been caused by conditions in the intestinal environment that were unfavorable to its stability, such as alkaline pH, pancreatin, and bile secretions71. After the intestinal phase, the presence or absence of phenolic compounds revealed the variable chemical behavior of phenolic compounds. For example, quercetin, which is extracted in relatively large amounts during gastric digestion, is not detected after intestinal digestion. A similar result was found in the study by Nignpense et al.70. This is consistent with evidence that quercetin has low solubility in alkaline media such as water and intestinal juice72.
The chemical characteristics of phenolic compounds, such as solubility, hydrophobicity, molecular weight, or even isomer configuration, are influencing their stability in the course of digestion73. The decrease of most phenolic compounds during in vitro digestion, regardless of the FRC extract microencapsulation method, may be related to the release of proteins and fibers by enzymatic degradation. Since both proteins and carbohydrates are able to interact with phenolic compounds and block their detection through spectrophotometric analysis68, 74. Changes in the simulated digestive environment’s pH can convert anthocyanins and transform some phenolics into different structural formulas with various chemical properties75.
The particle size of powder extracts has been associated with important characteristics such as susceptibility to deterioration, fluidity, appearance and dispersibility76. Particle size distribution graphs (Fig. 10S of the Supplementary Material) allowed to calculate for spray-dried and freeze-dried microcapsules average diameters (16.75 and 25.19 µm, respectively) within the values reported for spray-drying in general (10–100 µm)77, and for other microencapsulated natural products or by-products38, 47, 78, 79. The largest size of freeze-dried particles compared to the spray-dried ones may have been due to the low process temperature as well as the lack of strength to break frozen drops or to change surfaces during drying)80.
Figure 3 shows the scanning electron micrographs of CPF extracts microencapsulated by spray-drying (a) and freeze-drying (b). Microcapsules prepared by freeze-drying had a more deformed and irregular shape, with extensive wrinkles and a more toothed surface than the ones prepared by spray-drying, which had a spherical shape, few deformations, and a smooth surface, confirming the observations of other authors38, 50, 81.
Scanning electron micrographs of ciriguela peel extract microparticles prepared by (a) spray-drying and (b) freeze-drying.
According to Ballesteros et al.82, the quite different conditions of spray-drying and freeze-drying result in different morphology, shape, and size of microparticles. Moreover, superficial imperfections, such as wrinkles or cracks, occur when there is slow film formation during droplet microencapsulation83. Also, the type of encapsulating agent can influence the morphology of microcapsules. In fact, Bernstein and Noreña84 obtained similar particles with the toothed surface using gum arabic, likely due to sudden moisture loss during microencapsulation81. Even the deformations of microcapsules, i.e., irregular spherical shape, shrinks, and wrinkles on their surface, may have been due to the use of arabic gum as an encapsulating agent50.
Microcapsules placed in flexible laminated packages were stored for 90 days at 7 ± 1 °C, aiming at their use as an additive for low temperature stored products, and 25 ± 1 °C, representing the room temperature. Figure 4 shows the stability of atomized and lyophilized microcapsules under these conditions in terms of ability to retain their TPC content over time.
Stability analysis of spray-dried and freeze-dried microcapsules of ciriguela residue extract stored at 7 and 25 °C for 90 days in terms of ability to retain phenolic compounds. Different capital letters for microcapsules stored at the same temperature indicate statistically significant differences over time for the same powder. Different lowercase letters indicate statistically significant differences between powders obtained by the same encapsulation method and stored at different temperatures.
The freeze-dried microcapsules of CPF extract did not show any statistically significant difference in their TPC content (602.77–623.41 mg GAE g−1) (p > 0.05) after 90 days compared to the beginning (568.86 ± 0.98 mg GAE g1), regardless of the storage temperature. The spray-dried powder extract started with 476.82 ± 2.04 mg GAE g−1, and at the end of the stability at 7 °C it presented 485.34 ± 1.50 mg GAE g−1 and at 25 °C, 471.70 ± 2.47 mg GAE g−1, so there was no significant difference between the start and end time. This small increase in TPC after stability is due to recoveries and the formation of polyphenols as a consequence of the hydrolysis of conjugated polyphenols38, 85.
Spray-drying and freeze-drying proved to be suitable processes to prepare ciriguela peel extract microcapsules to be used as a source of phenolic compounds for foods, such as drinks, salty cookies, cookies, bakery products among others, as well as pharmaceuticals and cosmetics. The optimized conditions of microencapsulation by atomization were shown to be a temperature of 150 °C, a feed flow rate of 0.80 L h−1 and the use of 100% gum arabic as an encapsulating agent. The spray-dried extract showed higher TPC (486.82 mg GAE g−1). The phenolic compounds found in greater concentrations in the liquid extract of ciriguela residue were rutin, epicatechin gallate, chlorogenic acid, and quercetin, while rutin and myricetin were the most abundant in the atomized extract, and quercetin and kaempferol in the freeze-dried one. After simulated gastrointestinal digestion of microencapsulated extracts, rutin was the phenolic compound most abundant in microcapsules. Spray-dried and freeze-dried microcapsules, regardless of storage temperature (7 or 25 °C), maintained their high content of phenolic compounds almost unvaried for 90 days of storage.
The data used to support the findings of this study are available from the corresponding author upon request.
Augusto, P. E. D., Cristianini, M. & Ibarz, A. Effect of temperature on dynamic and steadystate shear rheological properties of siriguela (Spondias purpurea L.) pulp. J. Food Eng. 108(2), 283–289. https://doi.org/10.1016/j.jfoodeng.2011.08.015 (2012).
Barros, R. G. C., Andrade, J. K. S., Denadai, M., Nunes, M. L. & Narain, N. Evaluation of bioactive compounds potential and antioxidant activity in some Brazilian exotic fruit residues. Food Res. Int. 102, 84–92. https://doi.org/10.1016/j.foodres.2017.09.082 (2017).
Article PubMed CAS Google Scholar
Maldonado-Astudillo, Y. I. et al. Postharvest physiology and technology of Spondias purpurea L. and S. mombin L.. Sci. Hortic. 174, 193–206. https://doi.org/10.1016/j.scienta.2014.05.016 (2014).
Dutra, R. L. T. et al. Bioaccessibility and antioxidant activity of phenolic compounds in frozen pulps of Brazilian exotic fruits exposed to simulated gastrointestinal conditions. Food Res. Int. 100, 650–657. https://doi.org/10.1016/j.foodres.2017.07.047 (2017).
Article PubMed CAS Google Scholar
Macedo, D. A. et al. Bioaccessibility of phenolic compounds in native and exotic frozen pulps explored in Brazil using a digestion model coupled with a simulated intestinal barrier. Food Chem. 274, 202–214. https://doi.org/10.1016/j.foodchem.2018.08.099 (2019).
Insang, S., Kijpatanasilp, I., Jafari, S. & Assatarakul, K. Ultrasound-assisted extraction of functional compound from mulberry (Morus alba L.) leaf using response surface methodology and effect of microencapsulation by spray drying on quality of optimized extract. Ultrason. Sonochem. https://doi.org/10.1016/j.ultsonch.2021.105806 (2022).
Agudelo, C., Barros, L., Santos-Buelga, C., Martínez-Navarrete, N. & Ferreira, I. C. F. R. Phytochemical content and antioxidant activity of grapefruit (Star Ruby): A comparison between fresh freeze-dried fruits and different powder formulations. LWT - Food Sci. Technol. 80, 106–112. https://doi.org/10.1016/j.lwt.2017.02.006 (2017).
Shavandi , A. , Bekhit , AEA , Saeedi , P. , Izadifar , Z. & Khademhosseini , A. Polyphenol uses in biomaterials engineering .Biomaterials 167, 91–106.https://doi.org/10.1016/j.biomaterials.2018.03.018 (2018).
Article PubMed PubMed Central CAS Google Scholar
Renard, C. M. G. C. Extraction of bioactives from fruit and vegetables: State of the art and perspectives. LWT - Food Sci. Technol. 93, 390–395. https://doi.org/10.1016/j.lwt.2018.03.063 (2018).
Szabo, K., Catoi, A. F. & Vodnar, D. C. Bioactive compounds extracted from tomato processing by-products as a source of valuable nutrients. Plant Foods Hum. Nutr. 73, 268–277. https://doi.org/10.1007/s11130-018-0691-0 (2018).
Article PubMed CAS Google Scholar
Ben-Othman, S., Jõudu, I. & Bhat, R. Bioactives from agri-food wastes: Present insights and future challenges. Molecules 25(3), 510–544. https://doi.org/10.3390/molecules25030510 (2020).
Article PubMed PubMed Central CAS Google Scholar
Rezende, Y. R. R. S., Nogueira, J. P. & Narain, N. Comparison and optimization of conventional and ultrasound assisted extraction for bioactive compounds and antioxidant activity from agro-industrial acerola (Malpighia emarginata DC) residue. LWT - Food Sci. Technol 85, 158–169. https://doi.org/10.1016/j.lwt.2017.07.020 (2017).
Caldas, T. W. et al. Phenolic compounds recovery from grape skin using conventional and nonconventional extraction methods. Ind. Crops Prod. 111, 86–91. https://doi.org/10.1016/j.indcrop.2017.10.012 (2018).
Guandalini , BBV , Rodrigues , NP & Marczak , LDF Ultrasound-assisted sequential extraction of phenolics and pectin from mango peel.Food Res.Int.119 , 455–461.https://doi.org/10.1016/food.2018.12.011 (2019).
Article PubMed CAS Google Scholar
Ribeiro, A. M., Estevinho, B. N. & Rocha, F. Microencapsulation of polyphenols - The specific case of the microencapsulation of Sambucus Nigra L. extracts - A review. Trends Food Sci. Technol. 105, 454–467. https://doi.org/10.1016/j.tifs.2019.03.011 (2019).
Lee, Y. & Chang, Y. H. Microencapsulation of a maca leaf polyphenol extract in mixture of maltodextrin and neutral polysaccharides extracted from maca roots. Int. J. Biol. Macromol. 150, 546–558. https://doi.org/10.1016/j.ijbiomac.2020.02.091 (2020).
Article PubMed CAS Google Scholar
Aguilera-Chávez, S. L., Gallardo-Velázquez, T., Meza-Márquez, O. G. & Osorio-Revilla, G. Spray drying and spout-fluid bed drying microencapsulation of mexican plum fruit (Spondias purpurea L.) extract and its effect on in vitro gastrointestinal bioaccessibility. Appl. Sci. 12(4), 2213. https://doi.org/10.3390/app12042213 (2022).
Pudziuvelyte, L. et al. Microencapsulation of Elsholtzia ciliata herb ethanolic extract by spray-drying: Impact of resistant-maltodextrin complemented with sodium caseinate, skim milk, and beta-cyclodextrin on the quality of spray-dried powders. Molecules 24(8), 1461. https://doi.org/10.3390/molecules24081461 (2019).
Article PubMed PubMed Central CAS Google Scholar
Zhang, J., Zhang, C., Chen, X. & Quek, S. Y. Effect of spray drying on phenolic compounds of cranberry juice and their stability during storage. J. Food Eng. 269, 109744. https://doi.org/10.1016/j.jfoodeng.2019.109744 (2020).
Zanoni, F., Primiterra, M., Angeli, N. & Zoccatelli, G. Microencapsulation by spray-drying of polyphenols extracted from red chicory and red cabbage: Effects on stability and color properties. Food Chem. 307, 125535. https://doi.org/10.1016/j.foodchem.2019.125535 (2020).
Article PubMed CAS Google Scholar
Shishir, M. R. I. & Chen, W. Trends of spray drying: A critical review on drying of fruit and vegetable juices. Trends Food Sci. Technol. 65, 49–67. https://doi.org/10.1016/j.tifs.2017.05.006 (2017).
AOAC - Official Methods of Analysis of the Association of Official Analytical Chemists: Official Methods of Analysis of AOAC International. 21st Edition, AOAC, Washington DC (2019).
Wettasinghe, M. & Shahidi, F. Evening primrose meal: A source of natural antioxidants and scavenger of hydrogen peroxide and oxygen-derived free radicals. J. Agric. Food Chem. 47(5), 1801–1812. https://doi.org/10.1021/jf9810416 (1999).
Article PubMed CAS Google Scholar
Silva Júnior, M. E., Araújo, M. V. R. L., Santana, A. A., Silva, F. L. H. & Maciel, M. I. S. Ultrasound-assisted extraction of bioactive compounds from ciriguela (Spondias purpurea L.) peel: Optimization and comparison with conventional extraction and microwave. Arab. J. Chem. 14, 103260. https://doi.org/10.1016/j.arabjc.2021.103260 (2021).
Cai, Y. Z. & Corke, H. Production and properties of spray dried amaranthus betacyanin pigments. J. Food Sci. 65(7), 1248–1252. https://doi.org/10.1111/j.1365-2621.2000.tb10273.x (2000).
Cano-Chauca, M., Ramos, A. M. & Stringheta, P. C. Effect of the carriers on the microstructure of mango powder obtained by spray drying and its functional characterization. Innov. Food Sci. Emerg. Technol. 6(4), 420–428. https://doi.org/10.1016/j.ifset.2005.05.003 (2005).
Saénz, C., Tapia, S., Chávez, J. & Robert, P. Microencapsulation by spray drying of bioactive compounds from cactus pear (Opuntia ficus-indica). Food Chem. 114(2), 616–622. https://doi.org/10.1016/j.foodchem.2008.09.095 (2009).
Mahdavi, S. A., Jafari, S. M., Assadpoor, E. & Dehnad, D. Microencapsulation optimization of natural anthocyanins with maltodextrin, gum Arabic and gelatin. Int. J. Biol. Macromol. 85, 379–385. https://doi.org/10.1016/j.ijbiomac.2016.01.011 (2016).
Barbosa-Cánovas, G. V. & Juliano, P. Physical and chemical properties of food powders. In Encapsulated and Powdered Foods (ed. Onwulata, C.) (Taylor & Francis, 2005).
Caparino, O. A. et al. Effect of drying methods on the physical properties and microstructures of mango (Philippine ‘Carabao’ var.) power. J. Food Eng. 111(1), 135–148. https://doi.org/10.1016/j.jfoodeng.2012.01.010 (2012).
Brand-Williams, W., Cuvelier, M. E. & Berset, C. Use of a free radical method to evaluate antioxidant activity. Food Sci. Technol. 28, 25–30. https://doi.org/10.1016/S0023-6438(95)80008-5 (1995).
Sánchez-Moreno, C., Larrauri, J. A. & Saura-Calixto, F. A procedure to measure the antiradical efficiency of polyphenols. J. Sci. Food Agric. 76(2), 270–276. https://doi.org/10.1002/(sici)1097-0010(199802)76:2%3c270::aid-jsfa945%3e3.0.co;2-9 (1998).
Ramadan, M. F., Kroh, L. W. & Morsel, J. T. Radical scavenging activity of black cumin (Nigella sativa L.), coriander (Coriandrum sativum L.), and Niger (Guizotia abyssinica Cass.) crude seed oils and oil fractions. J. Agric. Food Chem. 51(24), 6961–6969. https://doi.org/10.1021/jf0346713 (2003).
Article PubMed CAS Google Scholar
Thaipong, K., Boonprakob, U., Crosby, K., Cisneros-Zevallos, L. & Byrne, D. H. Comparison of ABTS, DPPH, FRAP, and ORAC assays for estimating antioxidant activity from guava fruit extracts. J Food Compost. Anal 19(6–7), 669–675. https://doi.org/10.1016/j.jfca.2006.01.003 (2006).
Padilha, C. V. S. et al. Rapid determination of flavonoids and phenolic acids in grape juices and wines by RP-HPLC/DAD: Method validation and characterization of commercial products of the new Brazilian varieties of grape. Food Chem. 228, 106–115. https://doi.org/10.1016/j.foodchem.2017.01.137 (2017).
Article PubMed CAS Google Scholar
Dutra, M. C. P., Rodrigues, L. L., Oliveira, D., Pereira, G. E. & Lima, M. S. Integrated analyses of phenolic compounds and minerals of Brazilian organic and conventional grape juices and wines: Validation of a method for determination of Cu, Fe and Mn. Food Chem. 269, 157–165. https://doi.org/10.1016/j.foodchem.2018.07.014 (2018).
Article PubMed CAS Google Scholar
Rodríguez-Roque, M. J., Rojas-Graü, M. A., Elez-Martínez, P. & Martín-Belloso, O. Changes in vitamin C, phenolic, and carotenoid profiles throughout in vitro gastrointestinal digestion of a blended fruit juice. J. Agric. Food Chem. 61(8), 1859–1867. https://doi.org/10.1021/jf3044204 (2013).
Article PubMed CAS Google Scholar
Nunes, G. L. et al. Microencapsulation of freeze concentrated Ilex paraguariensis extract by spray drying. J. Food Eng. 151, 60–68. https://doi.org/10.1016/j.jfoodeng.2014.10.031 (2015).
Albuquerque , JG , Duarte , AM , Conceição , ML & Aquino , JS Integral utilization of seriguela fruit (Spondias purpurea L.) in the production of cookies.Rev. Fr.Bras.Frutic.https://doi.org/10.1590/0100-29452016229 (2016).
Reis, DS, Figueiro Neto, A., Ferraz, AV & Freitas, ST Production and conservation stability of dehydrated acerola flour at different temperatures.Braz.J. Food Technol.https://doi.org/10.1590/1981-6723.8315 (2017).
Leong, S. L., Pettersson, O. V., Rice, T., Hocking, A. D. & Schnürer, J. The extreme xerophilic mould xeromyces bisporus — growth and competition at various water activities. Int. J. Food Microbiol. 145(1), 57–63. https://doi.org/10.1016/j.ijfoodmicro.2010.11.025 (2011).
Damodaran, S. & Parkin, K. L. Fennema’s Food Chemistry (CRC Press, 2017).
Janiszewska-Turak, E. et al. The influence of carrier material on some physical and structural properties of carrot juice microcapsules. Food Chem. 236, 134–141. https://doi.org/10.1016/j.foodchem.2017.03.134 (2017).
Article PubMed CAS Google Scholar
Archaina, D., Leiva, G., Salvatori, D. & Schebor, C. Physical and functional properties of spray-dried powders from blackcurrant juice and extracts obtained from the waste of juice processing. Food Sci. Technol. Int. 24(1), 78–86. https://doi.org/10.1177/1082013217729601 (2017).
Article PubMed CAS Google Scholar
Kuck, L. S. & Norena, C. P. Z. Microencapsulation of grape (Vitis labrusca var. Bordo) skin phenolic extract using gum arabic, polydextrose, and partially hydrolyzed guar gum as encapsulating agents. Food Chem. 194, 569–576. https://doi.org/10.1016/j.foodchem.2015.08.066 (2016).
Article PubMed CAS Google Scholar
Nguyen, V. T., Tran, A. X. & Le, V. A. T. Microencapsulation of phenolic-enriched extract from cocoa pod husk (Theobroma cacao L.). Powder Technol. 386, 136–143. https://doi.org/10.1016/j.powtec.2021.03.033 (2021).
Rezende, Y. R. R. S., Nogueira, J. P. & Narain, N. Microencapsulation of extracts of bioactive compounds obtained from acerola (Malpighia emarginata DC) pulp and residue by spray and freeze drying: Chemical, morphological and chemometric characterization. Food Chem. 254, 281–291. https://doi.org/10.1016/j.foodchem.2018.02.026 (2018).
Article PubMed CAS Google Scholar
Tülek, Z. et al. Extraction optimization and microencapsulation of phenolic antioxidant compounds from lemon balm (Melissa officinalis L.): Instant soluble tea production. J. Food Process. Preserv. 45, e14995. https://doi.org/10.1111/jfpp.14995 (2020).
Bednarska, M. A. & Janiszewska-Turak, E. The influence of spray drying parameters and carrier material on the physico-chemical properties and quality of chokeberry juice powder. J. Food Sci. Technol. 57(2), 564–577. https://doi.org/10.1007/s13197-019-04088-8 (2020).
Article PubMed CAS Google Scholar
Daza, L. D. et al. Effect of spray drying conditions on the physical properties of Cagaita (Eugenia dysenterica DC.) fruit extracts. Food Bioprod. Process. 97, 20–29. https://doi.org/10.1016/j.fbp.2015.10.001 (2016).
Tsali, A. & Goula, A. M. Valorization of grape pomace: Encapsulation and storage stability of its phenolic extract. Powder Technol. 340, 194–207. https://doi.org/10.1016/j.powtec.2018.09.011 (2018).
Lourenço, S. C., Moldão-Martins, M. & Alves, V. D. Microencapsulation of pineapple peel extract by spray drying using maltodextrin, inulin, and Arabic gum as wall matrices. Foods https://doi.org/10.3390/foods9060718 (2020).
Shamaei, S., Seiiedlou, S. S., Aghbashlo, M., Tsotsas, E. & Kharaghani, A. Microencapsulation of walnut oil by spray drying: Effects of wall material and drying conditions on physicochemical properties of microcapsules. Innov. Food Sci. Emerg. Technol. 39, 101–112. https://doi.org/10.1016/j.ifset.2016.11.011 (2017).
Kaderides, K., Goula, A. M. & Adamopoulos, K. G. A process for turning pomegranate peels into a valuable food ingredient using ultrasound-assisted extraction and encapsulation. Innov. Food Sci. Emerg. Technol. 31, 204–215. https://doi.org/10.1016/j.ifset.2015.08.006 (2015).
Tran, T. T. A. & Nguyen, H. V. H. Effects of spray-drying temperatures and carriers on physical and antioxidant properties of Lemongrass leaf extract powder. Beverages 4(4), 84. https://doi.org/10.3390/beverages4040084 (2018).
Labuschagne, P. Impact of wall material physicochemical characteristics on the stability of encapsulated phytochemicals: a review. Food Res. Int. 107, 227–247. https://doi.org/10.1016/j.foodres.2018.02.026 (2018).
Article PubMed CAS Google Scholar
Ramírez, M. J., Giraldo, G. I. & Orrego, C. E. Modeling and stability of polyphenol in spray-dried and freeze-dried fruit encapsulates. Powder Technol. 277, 89–96. https://doi.org/10.1016/j.powtec.2015.02.060 (2015).
Comunian, T. A. et al. Assessment of production efficiency, physicochemical properties and storage stability of spray-dried chlorophyllide, a natural food colourant, using gum Arabic, maltodextrin and soy protein isolate-based carrier systems. Int. J. Food Sci. Technol. 46(6), 1259–1265. https://doi.org/10.1111/j.1365-2621.2011.02617.x (2011).
Caliskan, G. & Dirim, S. N. The effect of different drying processes and the amounts of maltodextrin addition on the powder properties of sumac extract powders. Powder Technol. 287, 308–314. https://doi.org/10.1016/j.powtec.2015.10.019 (2016).
Engels, C. et al. Characterization of phenolic compounds in jocote (Spondias purpurea L.) peels by ultra high-performance liquid chromatography/electrospray ionization mass spectrometry. Food Res. Int. 46(2), 557–562. https://doi.org/10.1016/j.foodres.2011.04.003 (2012).
Silva, R. V., Costa, S. C. C., Branco, C. R. C. & Branco, A. In vitro photoprotective activity of the Spondias purpurea L. peel crude extract and its incorporation in a pharmaceutical formulation. Ind. Crops Prod. 83, 509–514. https://doi.org/10.1016/j.indcrop.2015.12.077 (2016).
Kang, J., Liu, L., Liu, M., Wu, X. & Li, J. Antibacterial activity of gallic acid against Shigella flexneri and its effect on biofilm formation by repressing mdoH gene expression. Food Control 94, 147–154. https://doi.org/10.1016/j.foodcont.2018.07.011 (2018).
Santana Neto, D. C. et al. Solid–liquid extraction of bioactive compounds from Spondias mombin L. by-products: Optimization and identifcation of phenolic profle. Braz. J. Chem. Eng 39, 511–525. https://doi.org/10.1007/s43153-021-00209-z (2022).
Barbosa, P. O. et al. Açai (Euterpe oleracea Mart.) pulp dietary intake improves cellular antioxidant enzymes and biomarkers of serum in healthy women. Nutrition 32(6), 674–680. https://doi.org/10.1016/j.nut.2015.12.030 (2016).
Article PubMed CAS Google Scholar
Buniowska, M., Carbonell-Capella, J. M., Frigola, A. & Esteve, M. J. Bioaccessibility of bioactive compounds after non-thermal processing of an exotic fruit juice blend sweetened with Stevia rebaudiana. Food Chem. 221, 1834–1842. https://doi.org/10.1016/j.foodchem.2016.10.093 (2017).
Article PubMed CAS Google Scholar
Tomas, M., Toydemir, G., Boyacioglu, D., Hall, R. & Beekwilder, J. C. E. The effects of juice processing on black mulberry antioxidants. Food Chem. 186, 277–284. https://doi.org/10.1016/j.foodchem.2014.11.151 (2015).
Article PubMed CAS Google Scholar
González, R. L., Martos, M. V., Álvarez, J. A. P. & López, J. F. Changes in bioaccessibility, polyphenol profile and antioxidant potential of flours obtained from persimmon fruit (Diospyros kaki) co-products during in vitro gastrointestinal digestion. Food Chem. 256, 252–258. https://doi.org/10.1016/j.foodchem.2018.02.128 (2018).
Yucetepe, A., Altin, G. & Ozçelik, B. A novel antioxidant source: evaluation of in vitro bioaccessibility, antioxidant activity and polyphenol profile of phenolic extract from black radish peel wastes (Raphanus sativus L. var. niger) during simulated gastrointestinal digestion. Int. J. Food Sci. Technol. 56, 1376–1384. https://doi.org/10.1111/ijfs.14494 (2021).
Peanparkdee, M., Borompichaichartkul, C. & Iwamoto, S. Bioaccessibility and antioxidant activity of phenolic acids, flavonoids, and anthocyanins of encapsulated Thai rice bran extracts during in vitro gastrointestinal digestion. Food Chem. https://doi.org/10.1016/j.foodchem.2021.130161 (2021).
Nignpense, B. E., Latif, S., Francis, N., Blanchard, C. & Santhakumar, A. B. The impact of simulated gastrointestinal digestion on the bioaccessibility and antioxidant activity of purple rice phenolic compounds. Food Biosci. 47, 101706. https://doi.org/10.1016/j.fbio.2022.101706 (2022).
Podio, N. S., Baroni, M. V., P’Erez, G. T. & Wunderlin, D. A. Assessment of bioactive compounds and their in vitro bioaccessibility in whole-wheat flour pasta. Food Chem. 293, 408–417. https://doi.org/10.1016/j.foodchem.2019.04.117 (2019).
Article PubMed CAS Google Scholar
Gao, Y. et al. Formulation optimization and in situ absorption in rat intestinal tract of quercetin-loaded microemulsion. Colloids Surf. B 71(2), 306–314. https://doi.org/10.1016/j.colsurfb.2009.03.005 (2009).
Barba, F. J. et al. Bioaccessibility of bioactive compounds from fruits and vegetables after thermal and nonthermal processing. Trends Food Sci. Technol. 67, 195–206. https://doi.org/10.1016/j.tifs.2017.07.006 (2017).
Altin, G., Ayar, E. N. & Ozcelik, B. Phenolic-protein interaction: effects on functional properties of phenolics and advantages on delivery of phenolics. Glob. J. Med. Res. 19(L1), 1–11 (2019).
Kim, I., Moon, J. K., Hur, S. J. & Lee, J. Structural changes in mulberry (Morus Microphylla. Buckl) and chokeberry (Aronia melanocarpa) anthocyanins during simulated in vitro human digestion. Food Chem. 318, 126449. https://doi.org/10.1016/j.foodchem.2020.126449 (2020).
Article PubMed CAS Google Scholar
Botrel, D. A., Borges, S. V., Fernandes, R. V. B. & Do Carmo, E. L. Optimization of fish oil spray drying using a protein: Inulin system optimization of fish oil spray drying using a protein: inulin system. Dry. Technol. 32(3), 279–290. https://doi.org/10.1080/07373937.2013.823621 (2014).
Fang, Z. & Bhandari, B. Encapsulation of polyphenols - A review. Trends Food Sci. Technol. 21(10), 510–523. https://doi.org/10.1016/j.tifs.2010.08.003 (2010).
Figueiredo, J. A. et al. Encapsulation of camu-camu extracts using prebiotic biopolymers: Controlled release of bioactive compounds and effect on their physicochemical and thermal properties. Food Res. Int. 137, 109563. https://doi.org/10.1016/j.foodres.2020.109563 (2020).
Pang, S. F., Yusoff, M. M. & Gimbum, J. Assessment of phenolic compounds stability and retention during spray drying of Orthosiphon stamineus extracts. Food Hydrocoll. 37, 159–165. https://doi.org/10.1016/j.foodhyd.2013.10.022 (2014).
Saikia, S., Mahnot, N. K. & Mahanta, C. L. Optimisation of phenolic extraction from Averrhoa carambola pomace by response surface methodology and its microencapsulation by spray and freeze drying. Food Chem. 171, 144–152. https://doi.org/10.1016/j.foodchem.2014.08.064 (2015).
Article PubMed CAS Google Scholar
Tolun, A., Artik, N. & Altintas, Z. Effect of different microencapsulating materials and relative humidities on storage stability of microencapsulated grape pomace extract. Food Chem. 302, 125347. https://doi.org/10.1016/j.foodchem.2019.125347 (2020).
Article PubMed CAS Google Scholar
Ballesteros, L. F., Ramirez, M. J., Orrego, C. E., Teixeira, J. A. & Mussatto, S. I. Encapsulation of antioxidant phenolic compounds extracted from spent coffee grounds by freeze-drying and spray-drying using different coating materials. Food Chem. 237, 623–631. https://doi.org/10.1016/j.foodchem.2017.05.142 (2017).
Article PubMed CAS Google Scholar
Ré, M. I. Microencapsulation by spray drying. Dry. Technol. 16(6), 1195–1236. https://doi.org/10.1080/07373939808917460 (1998).
Bernstein, A. & Noreña, C. P. Z. Encapsulation of red cabbage (Brassica oleracea L. var. capitata L. f. rubra) anthocyanins by spray drying using different encapsulating agents. Braz. Arch. Biol. Technol. 58(6), 944–952. https://doi.org/10.1590/S1516-89132015060226 (2015).
Bakowska-Barczk, AM & Kolodziejczyk, PP Black currant polyphenols: Their storage stability and microencapsulation.Indian Crops Prod.34(2), 1301–1309.https://doi.org/10.1016/j.indcrop.2010.10.002 (2011).
This work was supported by the Coordination for the Improvement of Higher Education Personnel—Brazil (CAPES)—Finance Code 001;Authors are also acknowledged with the National Institute of Science and Technology of Tropical Fruits and National Council for Scientific and Technological Development (CNPq) for the financial support (Grant #309443/2019–9).
Food Science and Technology Graduate Program, Technology Center, Federal University of Paraíba, João Pessoa, Brazil
Marcony Edson da Silva Júnior, Ana Cristina Silveira Martins, Flávio Luiz Honorato da Silva & Maria Inês Sucupira Maciel
Laboratory of Physical-Chemical Analysis of Food, Department of Consumer Sciences, Federal Rural University of Pernambuco, Recife, Brazil
Maria Vitória Rolim Lemos Araújo
Department of Food Technology, Federal Institute of Sertão Pernambucano, Campus Petrolina, Rod. BR 407 Km 08, S/N, Jardim São Paulo, Petrolina, PE, 56314-520, Brazil
Department of Civil, Chemical and Environmental Engineering, Pole of Chemical Engineering, University of Genoa, Via Opera Pia 15, 16145, Genoa, Italy
Food Science and Technology Graduate Program, Federal Rural University of Pernambuco, Recife, Brazil
Maria Inês Sucupira Maciel
You can also search for this author in PubMed Google Scholar
You can also search for this author in PubMed Google Scholar
You can also search for this author in PubMed Google Scholar
You can also search for this author in PubMed Google Scholar
You can also search for this author in PubMed Google Scholar
You can also search for this author in PubMed Google Scholar
You can also search for this author in PubMed Google Scholar
M.E.S.J.: Conceptualization, Methodology, Writing, Investigation. M.V.R.L.A.: Investigation. A.C.S.M.: Investigation. M.S.L.: identification and quantification of the phenolic compounds by High-Performance Liquid Chromatography. F.L.H.S.: Formal analysis. A.C.: Writing-Review and Editing. M.I.S.M.: Supervision, Writing-Review and Editing.
Correspondence to Maria Inês Sucupira Maciel.
The authors declare no competing interests.
Springer Nature remains neutral with regard to jurisdictional claims in published maps and institutional affiliations.
Open Access This article is licensed under a Creative Commons Attribution 4.0 International License, which permits use, sharing, adaptation, distribution and reproduction in any medium or format, as long as you give appropriate credit to the original author(s) and the source, provide a link to the Creative Commons licence, and indicate if changes were made. The images or other third party material in this article are included in the article's Creative Commons licence, unless indicated otherwise in a credit line to the material. If material is not included in the article's Creative Commons licence and your intended use is not permitted by statutory regulation or exceeds the permitted use, you will need to obtain permission directly from the copyright holder. To view a copy of this licence, visit http://creativecommons.org/licenses/by/4.0/.
da Silva Júnior, M.E., Araújo, M.V.R.L., Martins, A.C.S. et al. Microencapsulation by spray-drying and freeze-drying of extract of phenolic compounds obtained from ciriguela peel. Sci Rep 13, 15222 (2023). https://doi.org/10.1038/s41598-023-40390-4
DOI: https://doi.org/10.1038/s41598-023-40390-4
Anyone you share the following link with will be able to read this content:
Sorry, a shareable link is not currently available for this article.
Provided by the Springer Nature SharedIt content-sharing initiative
By submitting a comment you agree to abide by our Terms and Community Guidelines. If you find something abusive or that does not comply with our terms or guidelines please flag it as inappropriate.
Scientific Reports (Sci Rep) ISSN 2045-2322 (online)
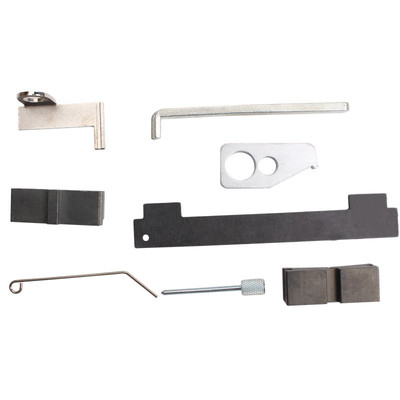
Sludge Dryer Sign up for the Nature Briefing newsletter — what matters in science, free to your inbox daily.